Spectrum Analyzer Specifications
When buying or determining what spectrum analyzer to use, it is necessary to understand its specifications
Spectrum Analyzer Tutorial Includes:
What is a spectrum analyzer
Spectrum analyzer types and technologies
Superheterodyne / sweep spectrum analyzer
FFT spectrum analyzer
Realtime spectrum analyzer
USB spectrum analyzer
Spectrum analyzer tracking generator
Specifications
Spectrum analyzer operation
Noise figure measurements
Phase noise measurements
Pulsed signal spectrum analysis
The spectrum analyzer specifications can be a little involved, but it is essential to have at least a basic understanding of them when selecting one of these test instruments.
Even when using a spectrum analyzer, understanding the specifications can ensure that its limitations are understood and the measurements that are made are ones within its capability.
Spectrum analyzers are expensive test instruments, it is essential that the best one is chosen for any applications. Understanding the basic specifications as well as the difference between analogue / superheterodyne spectrum analyzers, FFT spectrum analyzers and even real time spectrum analyzers can be important.
A little time studying the specifications can ensure that the correct test instrument is chosen.
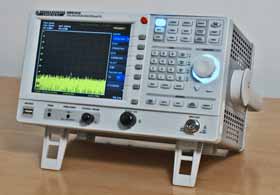
Spectrum analyzer types
Before delving into the specifications and what they actually means, one of the first steps is to select the right type of analyzer. There are several different types of analyzer, so it is essential to understand what each type is and what they are able to achieve.
- Superheterodyne spectrum analyzer: This type of spectrum analyzer uses the superheterodyne principle. A local oscillator converts the incoming signal down to a fixed frequency IF. By sweeping the local oscillator using a ramp voltage, it is possible to scan a range of frequencies. If the ramp voltage is also linked to the horizontal axis of the display and the vertical axis to the detected level of the signal, then a display of the spectrum is seen.
- FFT spectrum analyzer: The Fast Fourier Transform, FFT spectrum analyzer uses digital techniques. The incoming signal is sampled and successive samples are passed to an FFT processor to process the signal. The FFT processor provides all the signal processing so that spectrum information can be passed on to a control and display processor to be displayed.
- Real time spectrum analyzer : One of the issues with an FFT analyzer is that transient signals can be missed between successive samples for the FFT processor. To overcome this, a real time spectrum analyzer takes samples that overlap in time. In this way, and transient that occurs will be captured and can be analyzed. Real time spectrum analyzers are particularly useful for analyzing RF systems that are driven by processors as glitches and transients can occur. They are also very useful for capturing various forms of modulation and for frequency hopping systems.
- USB spectrum analyzer: Although USB spectrum analyzers are possibly not a different type of analyzer as such, they probably warrant a section as they provide a very cost effective way of creating a spectrum analyzer. By capturing the waveform and undertaking the processing in a specifically designed FPGA, the processed information can be passed to a computer over a USB interface to be displayed. This saves considerable cost and space.
Spectrum analyzer frequency coverage
Possibly one of the most important headline specifications for a spectrum analyzer is its frequency coverage.
Typically a spectrum analyzer will be able to measure from very close to zero Hertz right up to its top frequency.
Normally the bottom frequency limit is not an issue for most applications as RF spectrum analyzers are normally used for frequencies well into the RF spectrum. The lower limit may depend upon whether the test instrument is DC or AC coupled. DC coupling normally gives a much lower limit. A typical example of the lower limit for a high end spectrum analyzer may be around 2 Hz for DC coupling, but 10 MHz for AC coupling.
The advantage of AC coupling is that it removes any DC that may be present on the signal. If DC component is too large, then it could easily damage the input of the spectrum analyzer, and the repair could be expensive.
The main parameter required for the frequency coverage specification is the top limit. This should obviously include at least the fundamental of the signals of interest, but remember that spectrum analyzers are often required to measure spurious signals like intermodulation distortion and harmonics.
In order to be able to check the performance of any unit, module or circuit properly, it is necessary to see at least the third harmonic of the main signal, and preferably higher.
A careful judgement needs to be made of selecting the right top frequency, possibly with a certain amount of contingency. However increments in the top frequency tend to be fairly large and they come with a large cost increase.
Frequency accuracy specification
The frequency accuracy is an important specification for any analyzer. Although it is not a frequency counter, the frequency accuracy is often key to its specification.
The frequency accuracy is treated differently for the older superheterodyne swept analogue analyzers and the much newer FFT digitally based analyzers. It is worth taking a separate look at the specifications for both forms of test instrument separately. As the superheterodyne swept analyzer was the first on the scene, this will be addressed first:
- Analogue superheterodyne swept spectrum analyzers: The errors for this form of spectrum analyzer can be split into a number of different areas:
- Frequency reference inaccuracy: This error is determined primarily by the internal timebase oscillator within the analyzer. Today virtually all spectrum analyzers use a high performance crystal oven oscillator so this term is normally quite small. Also the internal architecture of the analyzer will also have a bearing on this term. However, when using a spectrum analyzer for any frequency measurements, it is worth remembering that the oven does take time to warm up and settle, so any measurements should only be taken once the analyse has settled. Full details for this will be given in the spectrum analyzer specification sheet.
- Span error: On older analyzers that may not have used digital techniques, a span error was also a key issue. This error was often split into two specs, based on the fact that many spectrum analyzers were fully synthesized for small spans, but are open-loop tuned for larger spans. Check out the operation of the analyzer, but for most modern ones this is not applicable
- Centre frequency error: Again, this form of error specification was applicable to older analyzers. In most cases it was much smaller than the span error.
- FFT based spectrum analyzers: The Fast Fourier Transform spectrum analyzers use a very different approach to achieving the same aim as the older test instruments. Within this group of analyzers, the real time spectrum analyzer is also included because it is really a specialised high performance version of the FFT spectrum analyzer. It is also possible to include the the USB spectrum analyzer as well because it operates using the same principles as the FFT analyzer - the only difference is that USB test instrument uses the display, display processing, controls, etc within a computer, whilst leaving the USB spectrum analyzer to undertake all the signal processing.
In these analyzers, all the reference signals, clocks and the like are derived from a high stability source. Often this is an oven controlled crystal oscillator - this could even be locked to a much higher standard source to give the system a much greater level of frequency accuracy. Any frequency measurement made by the analyzer will be fundamentally determined by the accuracy of the clock.
Typically frequency measurements are made using markers. A position on the screen is selected, and often this is a peak of a signal so that its centre frequency can be measured. It is mainly the frequency accuracy of these markers which is of interest.
There are several frequency accuracy specifications that are used within an FFT spectrum analyzer.
- Marker resolution: The marker resolution is not actually related to the frequency accuracy, but it gives the steps that the marker can make - it gives the step size between one position and the adjacent one. In many test instruments this can be as fine as 1 Hz. This is more than adequate, especially as some of the frequencies which modern analyzers can measure extend to many GHz.
- Marker frequency uncertainty: The marker uncertainty is what might be thought of as the accuracy of the system. As the markers give a readout of the frequency at which they are positioned, often giving the peak or centre frequency of a signal, it is this accuracy or more correctly the uncertainty which is of great importance.
The marker uncertainty figure consists of several elements. It can typically be determined as ± (marker frequency x reference accuracy + typically around 10% of resolution bandwidth + 0.5 x (span / (sweep points - 1) + marker resolution).
The frequency reference error can be calculated as ± (time since last adjustment x ageing rate + temperature drift + calibration accuracy).
The frequency accuracy of spectrum analyzers is not always easy to calculate in the laboratory, but today's high performance models will provide surprisingly high levels of accuracy, although using the simple calculations above, it is possible to gain a good estimate of the performance, without doing a full investigation of all the relevant parameters .
Phase noise specification
Phase noise has become increasingly important in recent years and along with this it is necessary to measure the phase noise performance of many oscillators and systems.
In order to make phase noise measurements, the performance of the spectrum analyzer must be better than that of the unit under test. If not the measurement will be that of the phase noise test instrument making the measurement because the phase noise from the spectrum analyzer would mask that of the unit under test.
In view of this, the phase noise performance of the analyzer is a key parameter.
Typically the phase noise specification is given as the level of single sideband noise that is measured when using a perfect signal source. It is specified as the level of phase noise measured in dBc (decibels relative to the carrier) measured in a 1Hz bandwidth at a given offset.
As the level of phase noise varies with the offset, the level may be specified at a number of frequencies, and a plot of the noise may also be given.
A typical specification may look something like the table below:
Offset from Carrier | Level |
---|---|
10 Hz | -80 dBc |
100 Hz | -108dBc |
1 kHz | < -125dBc |
10 kHz | < - 135 dBc |
100 kHz | < - 138 dBc |
1 MHz | < -145 dBc |
10 MHz | < - 154 dBc |
As the measurement reaches a 10 MHz offset, it is anticipated that the noise will remain constant, attaining the noise floor of the test instrument.
Amplitude accuracy specification
The spectrum analyzer specification for amplitude accuracy is of great importance for any measurements made by the test instrument.
There are two analyzer specifications associated with amplitude accuracy:
- Absolute accuracy specification: This spectrum analyzer specification refers to measurements where the absolute level is required. It may be a measurement of the power level of a signal expressed in terms of dBm, etc.
- Relative accuracy specification: The relative accuracy specification is slightly different. This specification is used when signals are expressed in terms of decibels when compared to another signal. For example a harmonic may be expressed in terms of decibels down on the carrier. These measurements are generally more accurate than the absolute measurements because the accuracy of the whole signal chain is
Resolution bandwidth specification
The resolution bandwidth specification for a spectrum analyzer is important when it is necessary to measure signals that are close together.
The resolution bandwidth is chiefly determined by the bandwidth of the filter used within the analyzer, but other factors like filter type, residual FM, and noise sidebands are factors to take into consideration when determining he useful resolution available.
More Test Topics:
Data network analyzer
Digital Multimeter
Frequency counter
Oscilloscope
Signal generators
Spectrum analyzer
LCR meter
Dip meter, GDO
Logic analyzer
RF power meter
RF signal generator
Logic probe
PAT testing & testers
Time domain reflectometer
Vector network analyzer
PXI
GPIB
Boundary scan / JTAG
Data acquisition
Return to Test menu . . .